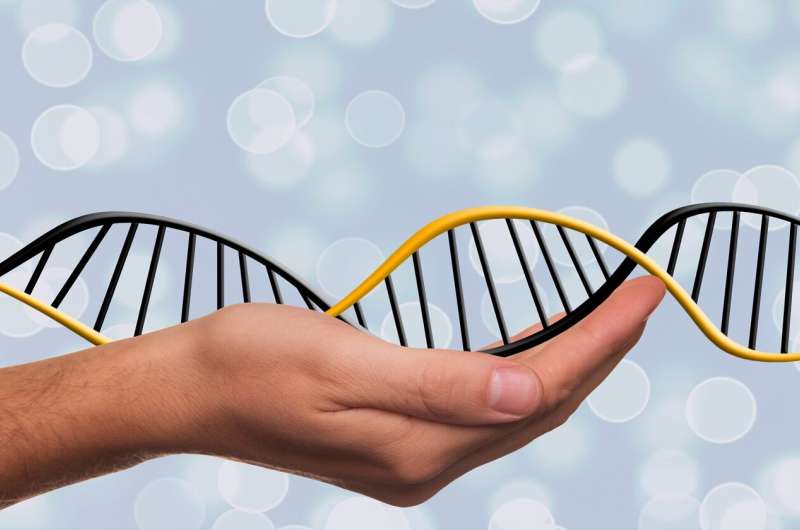
Credit: Pixabay/CC0 Public Domain
A research team led by André Marques at the Max Planck Institute for Plant Breeding Research in Cologne has revealed the profound effects of an atypical chromosome arrangement on genome organization and evolution. Your results will be published in the journal cell.
In every single cell in our body, our DNA, the molecule that carries the instructions for development and growth, is packaged along with proteins in structures called chromosomes. Complete sets of chromosomes together form the genome, the entire genetic information of an organism. In most organisms, including us, chromosomes appear as X-shaped structures when captured in their condensed, duplicated states in preparation for cell division. In fact, these structures are among the most iconic in all of science. The X shape is due to a narrowed region called the centromere, which serves to connect sister chromatids, which are identical copies formed by DNA replication of a chromosome. Most organisms studied are “monocentric,” meaning centromeres are confined to a single region on each chromosome. However, some animal and plant organisms exhibit a very different centromere organization: instead of a single constriction as in the classic X-shaped chromosomes, chromosomes in these organisms harbor multiple centromeres arranged in a line from one end of a sister chromatid to the other. Hence, these chromosomes lack a primary constriction and the X-shape, and species with such chromosomes are known as “holocentric,” from the ancient Greek word hólos, meaning “whole.”
A new study led by André Marques from the Max Planck Institute for Plant Breeding Research in Cologne now shows the remarkable impact of this non-classical type of chromosome organization on genome architecture and evolution.
To determine how holocentricity affects the genome, Marques and his team used high-precision DNA sequencing technology to decode the genomes of three closely related holocentric beaked sedges, grass-like flowering plants found worldwide and often the first to invade new habitats. For reference, the team also decoded the genome of their closest monocentric relative. Therefore, comparing the holocentric beaked sedges with their monocentric relatives allowed the authors to attribute any observed differences to the effects of holocentricity.
Their analyzes show striking differences in genome organization and chromosome behavior in holocentric organisms. They found that centromere function is distributed across hundreds of small centromere domains in holocentric chromosomes. While in monocentric organisms the genes are largely concentrated far away from centromeres and their immediate surroundings, in holocentric species they are evenly distributed over the entire length of the chromosomes. Furthermore, chromosomes in monocentric species are known to mix to a high degree during cell division, a property that appears to play a role in the regulation of gene expression. Notably, these long-range interactions were greatly reduced in the beaked sedges with holocentromeres. Therefore, holocentricity fundamentally affects genome organization as well as the behavior of chromosomes during cell division.
In holocentric organisms almost any given chromosome fragment harbors a centromere and therefore has proper centromere function, which is not the case in monocentric species. In this way, holocentromeres were thought to stabilize chromosomal fragments and fusions, thus promoting rapid genome evolution or an organism’s ability to make instantaneous, large-scale changes to its DNA. In one of the beaked sedges they analyzed, Marques and his team were able to show that chromosome fusions facilitated by holocentromeres allowed this species to maintain the same number of chromosomes even after quadrupling the entire genome. In another beaked sedge they analyzed, a species with just two chromosomes, the lowest of any plant, holocentricity was found to be responsible for the dramatic reduction in chromosome count. Therefore, holocentric chromosomes can enable the formation of new species through rapid evolution at the genome level.
According to Marques, “Our study shows that the transition to holocentricity has profoundly affected the way genomes are organized and regulated, and that genomes can evolve rapidly through the merging of their chromosomes.” The team’s findings also show exciting implications for plant breeding, which typically relies on the ability to exchange DNA and genes between chromosomes and organisms. “Holocentric plants allow for DNA swapping near centromeres, something that is usually suppressed in monocentric species. By understanding how holocentrics do this, we could ‘decode’ these genes in monocentric species and make them more amenable to breeding. higher-performing, hardier plant species.”
The protein complex SMC has been shown to ensure the dynamics of holocentromeres
Paulo G. Hofstatter et al, Repeat-based holocentromeres influence genome architecture and karyotype evolution, cell (2022). DOI: 10.1016/j.cell.2022.06.045
cell
Provided by the Max Planck Society
Citation: Speeding up evolution at the genome level by alternative chromosome configuration (2022, August 4), retrieved August 4, 2022 from https://phys.org/news/2022-08-evolution-genome-alternative-chromosome-configuration .html
This document is protected by copyright. Except for fair trade for the purpose of private study or research, no part may be reproduced without written permission. The content is for informational purposes only.
#Accelerating #evolution #genome #level #alternative #chromosome #configuration
Leave a Comment