Summary: Researchers show how the anterior cingulate cortex and motor cortex work together to update behavior when a new step is added to an established task.
Source: WITH
Life is full of processes to be learned and then re-learned as they become more sophisticated. One day you just log into an app with a password, the next day you also need a code that will be sent to you via SMS. One day you might just pop your favorite microwavable food in the oven for six minutes, but then the packaging changes and you have to cook it for three minutes, stir it, and then heat it for another three minutes. Our brain needs a way to keep up.
A new study by neuroscientists at MIT’s Picower Institute for Learning and Memory reveals some of the circuitry that helps a mammalian brain add steps.
in the nature communication The scientists report that when they changed the rules of a task and the rats had to adapt from just one step to two, a pair of regions on the brain surface, or cortex, worked together to update this understanding and change the rats’ behavior to adapt to the new regime.
The anterior cingulate cortex (ACC) seemed to recognize when the rats weren’t doing enough and updated cells in the motor cortex (M2) to adjust task behavior.
“I started this project about 7 or 8 years ago when I wanted to study decision making,” says Daigo Takeuchi, a researcher at the University of Tokyo who led the postdoctoral work at the RIKEN-MIT Laboratory for Neural Circuit Genetics at The Picower Institute, led by senior author and Picower Professor Susumu Tonegawa.
“Recent studies found a role for M2. I wanted to investigate what upstream circuitry affects this.”
Stumble up the second step
Takeuchi and Tonegawa traced neural circuit connections leading to M2 and found that many originated in the ACC. They began to recognize the ACC’s role in guiding M2’s sequential decisions when they introduced genetic manipulation into ACC cells that enabled them to repress their activity. This “chemogenetic” deactivation of the ACC had a very specific effect.
When the task rules changed so that rats not only had to poke their snout in one hole to get a small reward, but had to poke their nose in a sequence of two holes, it took the rodents with silenced ACCs much longer to see the rule change to recognize .
They did not realize that the second sting was necessary for much longer than rats with normal ACC activity. However, rats had no trouble going from two steps back to just one step, regardless of whether their ACC was muted.
When the scientists chemically-genetically silenced the terminals of the ACC cells in M2, they obtained the same results as silencing the ACC altogether. They also silenced other areas of the cortex, but this did not affect the rats’ ability to notice and adapt to the rule change.
Taken together, these manipulations confirmed that it was specifically the ACC’s connections to M2 that helped the rats notice and adjust to the change from one step to two steps.
But what effect does the ACC have in the M2? Takeuchi and his co-authors measured the electrical activity of cells in M2 while the rats played their nose-poking, rule-changing game. They found that many cells were particularly activated by different task rules (e.g. one step or two steps). However, when they silenced the ACC, it squelched that rule selectivity.
Within M2, Takeuchi and the team also noticed populations of neurons that responded preferentially to positive outcomes (reward for performing the task correctly) and negative outcomes (no reward for performing the task incorrectly).
They found that silencing the ACC actually increased the activity of negative outcome-encoding neurons during negative feedback, particularly in the first 10-20 rounds after the rules were changed from one step to two. This correlated strongly with the timing, or “epoch,” of the rats’ worst performance.
“It seems likely that the epoch-specific disruption of the animals’ second-choice performance is associated with the excessive increase in activity of negative outcome-activated neurons caused by ACC silencing,” they wrote in the study.
The team further confirmed that the feedback or results phase matters by using a different technique to mute the ACC. By engineering ACC neurons to be suppressed by flashes of light (a technique called “optogenetics”), they were able to precisely control when the ACC went offline.
They found that if they did so after the rats made a wrong choice when the rules switched from one bump to two, they could cause the rats to keep making mistakes. Optogenetic silencing of the ACC after the rats made a correct choice did not affect their subsequent behavior.
“These results indicate that ACC neurons process error feedback information after an erroneous second response and use this information to adjust the animal’s sequential selection responses in subsequent trials,” they wrote.
A threshold that is too high
The evidence painted a clear picture: when the rats realized that an extra step was now required, the ACC’s job was to learn from negative feedback and signal M2 to take the second step. If the ACC was not available when the feedback was given, then M2 cells, which obviously emphasize negative outcomes, would become particularly active and the rats would not take the required second step for a while before finally getting the hang of it.
Why would less ACC activity somehow increase the negative result encoding the activity of the cells in M2? Takeuchi hypothesizes that the ACC actually stimulates inhibitory cells in M2 that normally modulate the activity of these cells. With reduced ACC activity, the negative results encoding M2 cells experience less inhibition.
See also
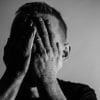
The behavioral outcome, he theorizes, is that the rats therefore need more evidence of the rule change than they should. The mechanism isn’t entirely clear, Takeuchi conceded, but the rats appear to need more time to experience outcome feedback if they make the right decision to take a second step before they’re convinced they’re up are on the right track.
Takeuchi said that while the results show the circuitry needed to adapt to a rule change that requires more steps in a process, they also raise some interesting new questions. Is there another circuit to notice when a multi-step process has become a single-step process? If so, is this circuit integrated with the one discussed in this study? And if the threshold model is correct, how exactly does it work?
The implications are not only important for understanding the neural basis of natural sequential decisions, but could also be important for AI applications such as games or industrial work, each of which can involve tasks with multiple steps.
About this news from neuroscientific research
Author: press office
Source: WITH
Contact: Press Office – MIT
Picture: Image is attributed to Tonegawa Lab/MIT Picower Institute
Original research: Open access.
“Cingulate-motor circuits update rule representations for sequential voting decisions” by Daigo Takeuchi et al. nature communication
abstract
Cingulate motor circuits update rule representations for sequential selection decisions
The anterior cingulate cortex mediates the flexible updating of an animal’s selection responses to regulatory changes in the environment. However, how the anterior cingulate cortex drives the motor cortex to reorganize rule representations and generate the required motor outputs remains unclear.
Here we show that chemogenetic silencing of the terminal projections of cingulate cortical neurons in the rat secondary motor cortex disrupts choice performance in studies immediately after rule switching, suggesting that these inputs are necessary to update rule representations for choice decisions stored in the motor cortex. In fact, silencing of the cingulate cortex decreases the rule selectivity of secondary motor cortical neurons.
Furthermore, optogenetic silencing of cingulate cortical neurons, timed to error attempts immediately after the rule change, exacerbates errors in subsequent attempts.
These results suggest that the cingulate cortex monitors behavioral errors and updates rule representations in the motor cortex, demonstrating a critical role for cingulate motor circuits in adaptive choice behavior.
#task #adds #steps #circuitry #helps #notice #Neuroscience #News
Leave a Comment